Supramolecular Polymers
1. Bioinspired Fuel-driven Living and Transient Supramolecular Polymerization
Biological systems host complex adaptive networks with precise Spatio-temporal control, coupled with sophisticated functionality. In the recent past, our group has made significant inroads towards elucidating the synthetic strategies for the realization of these concepts in materials. Extracting the gist of out-of-equilibrium, fuel-driven networks from the biological world, chemical fuel-driven supramolecular polymerization strategies pioneered in our research laboratory have notably advanced the frontiers of constructing controllable and adaptive materials with mono-disperse structure and predictive sequences. The following are some of the strategies used by us towards achieving adaptive, autonomous, and like-life out-of-equilibrium supramolecular materials.
ATP-fuelled, enzymatically Controlled Approach
Inspired from Actin protein assemblies, which shows controlled polymerization in response to adenosine triphosphate (ATP) fuel via nucleation-elongation process in a temporal manner to regulate mechanical stability of cell, we have introduced ATP selective and ATP-fuelled synthetic strategies to controlled supramolecular polymerization of a phosphate receptor functionalized monomer (dormant monomer). Here, the system undergoes fuel-driven nucleation and seeded growth that provide length control and narrow dispersity of the resultant assemblies. Furthermore, coupling via ATP-hydrolysing enzymes yielded its transient characteristics. Such control originates from growth kinetics to dimensional control on one end, to lifetime of materials on the other. We believe this temporally controlled living (with active chain termini) and transient self-assembly approach not only is a conceptual synonym to a much bigger and complex biological relative, but it also provides primed precursors of complex active materials to be developed in the years to come.
Chemical Reaction-driven Strategies
Recently, we developed a common synthetic strategy that works on biological principles such as chemical fuel-driven control over structural and temporal self-assembly profile. We employ simple yet powerful dynamic covalent bond chemistry, where covalent bonds are being made and broken by synthetic means only, to kinetically control the formation of supramolecular polymer. Here, we apply chemical reaction(s) to activate building blocks to trigger their assembly in a kinetically controlled manner, later returning back to the original state under the same reaction conditions. In our recent work we explored the pH responsive imine chemistry where the design involves the conversion of non-assembling CT complex (inactive/dormant state) between aromatic donor and benzaldehyde substituted viologen acceptor into a self-assembling CT amphiphile (active state) via an in situ kinetically controlled imine bond formation with an alkyl amine (fuel) similar to inactive and active states of protein monomers in biological assemblies which can be triggered by a fuel. Further, in our biomimetic approach, we deployed two orthogonal enzymatic reaction networks to create adaptive, autonomous, and like-life out-of-equilibrium supramolecular materials.
Redox-fuelled Systems
ver the years this highly competitive and active area of research has witnessed seminal works from leading groups around the world. However, their applied strategies remain specific to systems and inherent molecular structures as the entailing characteristics such as mechanism, energetic parameters, and monomer exchange dynamics are significantly diverse among various classes of supramolecular polymers. In this context, the redox chemistry mediated approaches introduced by us provide a general strategy applicable to a wide domain of monomers and materials. For example, our recent approach is reminiscent of well-known reversible-deactivation radical polymerization methods (also known as living radical polymerization) such as ATRP, NMP, RAFT, etc. in the field of polymer chemistry for the controlled synthesis of covalent polymers with precise degree of polymerization, dispersity and sequence.
Hence, this fuel-driven supramolecular polymerization ushers a new area in supramolecular chemistry whereby researchers could start to apply similar tricks to those that ATRP/NMR/RAFT polymer chemists frequently apply, to create multi-component dynamic materials with precision and sequence.
2. Multicomponent Supramolecular Polymerization
Creating a general strategy, applicable to a large class of molecules, for controlled aggregation effectively impacts its functional aspects as well. The structural and sequence control brings areas such as energy transfer, catalysis, chemical signalling into the domain of temporal control, opening up possibilities that photonic materials, organo-catalysts, electronically active materials and drug delivery platforms could be potentially designed with precise structure and temporally executed, thus mimicking the adaptive characteristics of biological world. In addition, this development is expected to have a deeper impact on soft-lithography of organic nanostructures as kinetically controlled processes adding finesse to device fabrication. Control over the temporal profile of material growth allows unprecedented sequence control of supramolecular aggregates leading to fabrication of complex, multicomponent structures such as, the exotic and highly coveted, axial p-n organic hetero-junctions and heterostructures at nanoscale and over the length scale of supramolecular polymers. Recently, we have shown kinetic and thermodynamically controlled approaches for the design of axial organic heterostructures, via the supramolecular block co-polymerization of opto-electronically active organic semiconducting monomers.
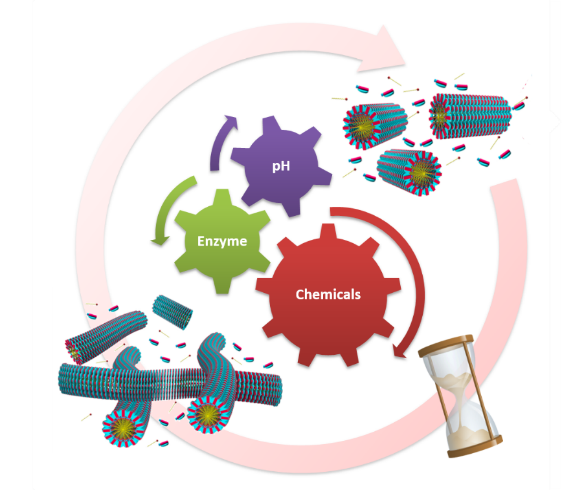
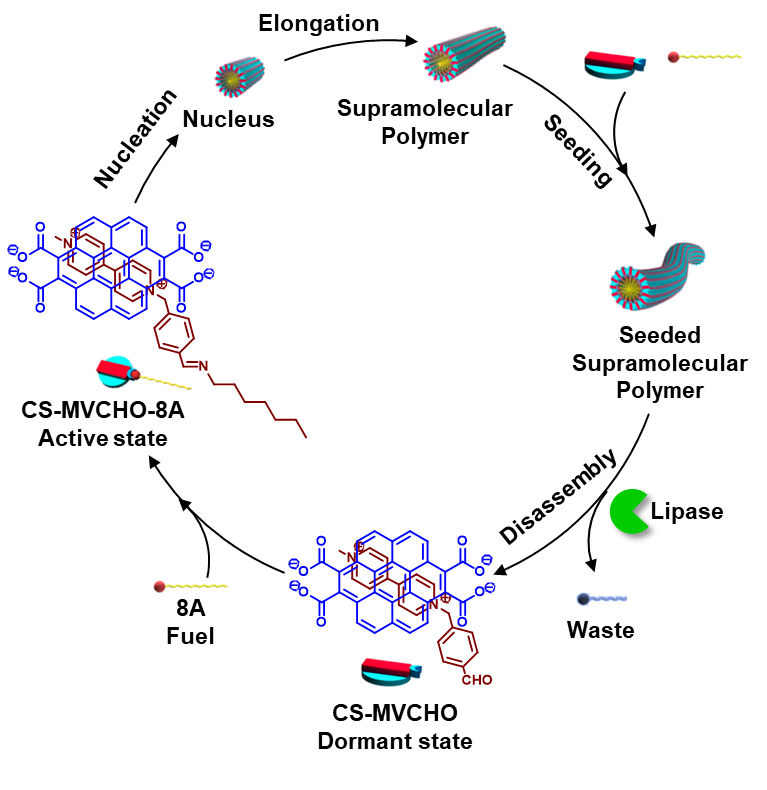
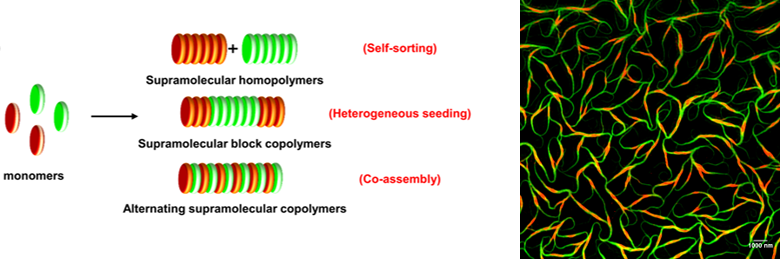
Angew. Chem. Int. Ed. 2020, https://doi.org/10.1002/anie.202006614
Chem. Commun. 2020, 56, 1505–1508;
Bull. Chem. Soc. Jpn. 2018, 91, 687–699;
Nat. Commun. 2018, 9, 1295;
Nat. Commun. 2014, 5, 5793. (Highlighted in Nature Chemistry, Nature India)
J. Am. Chem. Soc. 2017, 139, 16568. (JACS Front Cover, Spotlight Article)
Angew. Chem. Int. Ed. 2017, 129, 1349. (Hot Article, Highlighted in Nature Nanotechnology)
Chem. Sci. 2020
Nat. Commun. 2019, 10, 450.
Nat. Commun. 2020, 11, 3967.
ACS Appl. Mater. Interfaces 2020, 12, 5259–5264.
ChemSystemsChem 2019, 1, e1900042;
Chem. Sci. 2017, 8, 6030. (Hot Article, Chem. Sci. Blog)
Angew. Chem. Int. Ed. 2020, https://doi.org/10.1002/anie.202006248
J. Am. Chem. Soc. 2020, https://doi.org/10.1021/jacs.0c04404
J. Am. Chem. Soc. 2020, 142, 7606–7617.
Angew. Chem. Int. Ed. 2017, 56, 13767.
- Back to previous page
- |
-
Page last updated date:05-06-2025 04:57 PM